Computer Model Reveals Underlying Mechanism of Hemoglobin S Fibers in Sickle Cell Disease
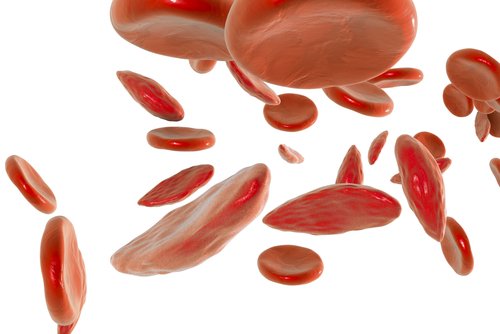
Researchers at Brown University have developed computer models that recreate what happens with hemoglobin molecules inside red blood cells affected by sickle cell disease.
Their study, “Mesoscopic Adaptive Resolution Scheme toward Understanding of Interactions between Sickle Cell Fibers,” appeared in the Biophysical Journal. It describes a virtual model authors hope will help develop better targeted therapies to fight sickle cell disease.
A genetic disorder, sickle cell disease is characterized by abnormally shaped red blood cells. Its patients cannot produce normal hemoglobin — the compound that binds oxygen and transports it throughout the body. Instead, they produce hemoglobin S, which forms long fibers that alter the shape of red blood cells into a crescent form.
These sickle red blood cells are very fragile, breaking down prematurely; this leads to anemia. They also form clots in small blood vessels, inducing painful episodes known as vaso-occlusive crisis or sickle cell crisis.
“The goal of our work is to model both how these sickle hemoglobin fibers form as well as the mechanical properties of those fibers,” Lu Lu, a student at Brown’s Division of Applied Mathematics and the study’s lead author, said in a news release. “There had been separate models for each of these things individually developed by us, but this brings those together into one comprehensive model.”
Researchers based their new proposed model on detailed biomechanical information about how hemoglobin S molecules behave and bind to each other. Despite the model’s overwhelming amount of data, they were able to simulate by computer all the underlying process of fiber formation and growth.
To do this, researchers had to use what they call a mesoscopic adaptive resolution scheme, or MARS. This let them analyze the initial fiber formation mechanism in detail, but also gave them a general view of the fiber growth mechanism. MARS allowed the model to retain crucial information on how the fibers behave mechanically, while blurring out the fine details of each hemoglobin molecule.
“By eliminating the fine details where we don’t need them, we develop a model that can simulate this whole process and its effects on a red blood cell,” said George Karniadakis, professor of applied math at Brown and the paper’s senior author.
With this simulation model, the research team was able to find out how hemoglobin S fibers are formed inside a red blood cell and produce the different shapes that can be observed in patients.
The model can now be used to test in advance new potential therapies, and pre-determine their efficacy and potency in disrupting hemoglobin fibers. It can also aid in finding new potential therapeutic targets that until now were unrecognized.
“The models give us a way to do preliminary testing on new approaches to stopping this disease,” Karniadakis said. “Now the goal is to use these models to look for ways of preventing disease onset.”