New model developed to track inflammation-driven blood clots
Tool may help scientists better assess the effectiveness of SCD treatments
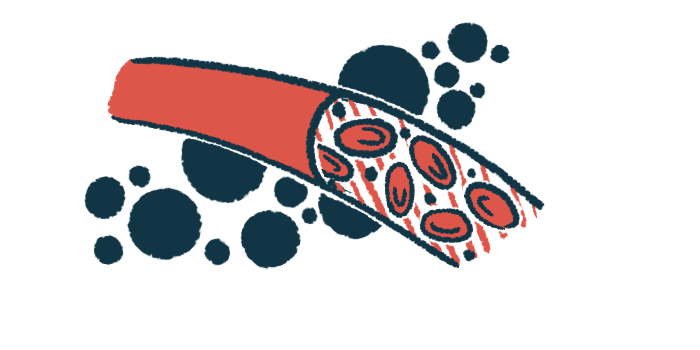
Researchers have developed a modelling system of tiny blood vessels to track inflammation-driven blood clot formation that can cause complications in sickle cell disease (SCD) and other conditions.
“One of the biggest things about being able to create [blood clots] under inflammatory conditions is that we are now able to map it out over time and develop hypotheses about which drugs would be most helpful, in which context, and importantly, when,” Wilbur Lam, MD, PhD, a professor at Emory University and Georgia Tech, and clinician at Children’s Healthcare of Atlanta, said in a university news story.
The development and testing of the model were detailed in a study, “Clinically relevant clot resolution via a thromboinflammation-on-a-chip,” published in the journal Nature.
Coagulation, or clotting, is a critical process that stops bleeding after blood vessel damage. It involves the clumping of small cell fragments known as platelets and the formation of a mesh made up of a protein called fibrin. Once the injury heals, clots are dissolved in a process called fibrinolysis.
In SCD, red blood cell sickling is caused by the production of an abnormal version of hemoglobin, the protein that carries oxygen in these cells. This can lead to red blood cell destruction, or hemolysis, and painful vaso-occlusive crises (VOCs), whereby sickled red blood cells obstruct blood vessels and block blood flow, damaging tissues and organs.
Thromboinflammation implicated in sickle cell disease
Recent studies have implicated thromboinflammation in SCD, in which hemolysis and VOCs can trigger inflammation-driven blood clot formation, resulting in various complications, further organ damage, and an elevated mortality risk. Thromboinflammation has also been shown to occur in cardiovascular diseases, such as heart attack and stroke.
However, what drives thromboinflammation and its resolution remains poorly understood due to the small size of the affected blood vessels. Moreover, studying this process in animals is challenging because it can take weeks to months to resolve.
“Animal models are challenging,” said Lam, the study’s corresponding author, who also noted it’s “extremely difficult to closely observe a clot in a specific part of a mouse for weeks.”
Therefore, alternative models that better recapitulate clot formation and resolution processes are urgently needed.
To address this, Lam and his colleagues developed a thromboinflammation-on-a-chip model that can sustain blood clots for several months using human blood in a human vein. The system accurately mimics clot formation and their natural resolution, and can be used to assess the effectiveness of several treatments.
“Our practical measure is so unique because it is hard to study thrombi [blood clots] in clinical settings due to the low spatial resolution of existing tools, so it’s harder to clearly see how these clots resolve,” said Yongzhi Qiu, PhD, the study’s first author and an assistant professor at Emory University School of Medicine.
Using this system, the team mapped out the distinct phases of clot formation and resolution. A pro-inflammatory signaling protein called TNF was infused into the system to induce inflammation. Then, whole blood was pumped into the inflamed blood vessels to trigger clot formation, which was marked by the clumping of platelets, the formation of fibrin, and the recruitment of immune neutrophils. A detailed analysis also showed inflammation shifted the balance of fibrinolysis in favor of blood clot formation.
“Because we created this system where we observe a blood clot on a chip over long periods of time, such as days to weeks, we can map out its entire resolution process, and that has never been done before,” Lam said.
Blood clot resolution happened in 3 stages
Blood clot resolution then happened in three stages marked by a decrease in the size of platelet clumps, followed by fibrin degradation, and removal of the remaining clot components. Experiments showed neutrophils were responsible for early clot resolution by releasing an enzyme called neutrophil elastase, which can degrade fibrin, but is also known to cause tissue damage.
“The function of neutrophil elastase in the context of clot resolution appears to be a double-edged sword that needs to be balanced to favour fibrin degradation over tissue damage,” the team wrote.
The system was then exposed to tissue plasminogen activator, or tPA, a so-called clot-busting therapy used in the acute management of heart attacks and strokes. The therapy removed nearly all the deposited fibrin and most recruited neutrophils and platelets overnight. In addition, tPA facilitated blood vessel repair, an effect that was previously unknown, according to the scientists.
To mimic what happens in SCD, whole blood from SCD patients was infused into the system without being exposed to TNF. This was sufficient to trigger significant clot formation, which gradually resolved over time after the infusion of healthy blood. By contrast, using healthy blood instead of SCD blood had no such effect on blood clotting.
The anticoagulant enoxaparin was then added to SCD blood to block fibrin formation and to investigate the role of platelet clumps and neutrophils. The team noted that neutrophil-platelet aggregates were present in SCD blood, some of which were big enough to block blood flow. When Adakveo (crizanlizumab-tmca), a medicine approved to reduce the frequency of VOCs in SCD patients, was added, the neutrophil-platelet aggregates were significantly reduced.
Targeting coagulation factors and neutrophil-platelet interactions may mitigate VOCs in SCD, the team noted.
“Our model is ideally suited to answer specific questions about the underlying mechanisms of [disease development] and mechanisms of action of drugs that cannot be evaluated [in living animals],” the researchers wrote.